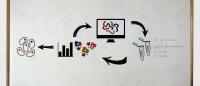
Dancing with Protein
Life’s molecular machinery operates by hidden rules. Uncovering them could open new doors to new medicines and biotechnologies.
There is no television in Dr. Anum Glasgow’s living room. Instead, there’s a whiteboard. This way, if a novel idea about designing technologically valuable proteins the world hasn’t yet seen comes to her at home–rather than her laboratory in Columbia’s Department of Biochemistry and Molecular Physics–she can readily explore it.
“We are trying to understand, predict and control the shapes and functions of proteins,” she says, speaking for the team of postdocs, graduate and undergraduate students she assembled in pandemic times since starting her job as Assistant Professor in early 2022. “We want to do a kind of protein engineering that still is not possible.”
Think here about decoy proteins – receptor traps– that capture viruses before they can bind to receptors on cells and gain entry into the body. Think of enzymatic proteins that deconstruct plastics to their chemical building blocks, which then can be reused. Now you are thinking like Dr. Glasgow.
Her first contact with science was in a highschool biology project in which she studied territorial behaviors in crayfish. But she only realized science was an actual career option when she was an undergraduate at Johns Hopkins University. There, she joined a chemical engineering laboratory that was developing techniques for fabricating tiny, flat metallic templates that could self-fold into minuscule three-dimensional shapes like boxes.
“We were thinking about devices for delivering drugs and robotic surgery,” she says.
But it was the overall strategy of getting two-dimensional structures to self-assemble into functional three-dimensional objects that she couldn’t stop thinking about. “Of course, I found my way to proteins,” she says.
That natural segue from metallic structures is anchored in a seminal feature of proteins: They start as linear sequences of amino-acid building blocks, which self-fold into 3D proteins with biological functions ranging from regulating sugar levels (insulin) to expressing genes (RNA polymerase).
Dr. Glasgow’s journey into the universe of proteins intensified as a graduate student in Dr Danielle Tullman-Ercek’s lab at the University of California, Berkeley. There, she studied the injectisome. That’s the nickname for a bacterial protein system (type III secretion system) that injects proteins into cells where those proteins reconfigure into shapes, or conformations, that can wreak havoc.
“We weren’t focused on the system’s native functions,” Dr. Glasgow notes. “We were trying to repurpose it to manufacture biopolymers,” including ones useful as adhesives and biodegradable plastics. “I wanted to learn how to do protein design, so we could rationally design proteins to do new things.”
She quickly learned how hard it is to design proteins from scratch. Each protein’s behaviors and capabilities, for one, reside in the ensemble of many shapes it can assume. This already daunting complexity magnifies when considering allosteric effects. Those are changes in protein structure and function due to interactions between the protein and other molecules.
Getting a better handle on these structural dynamics of proteins was a primary goal during Dr. Glagow’s postdoc years in the lab of Tanja Kortemme, PhD, at the University of California, San Francisco. During that time, Dr. Glasgow mastered techniques for generating huge libraries of protein variants in a process of directed evolution. Powerful as this approach is, it can typically only improve on a protein’s existing function, but not lead to proteins with entirely new capabilities.
In her work in the Kortemme Lab, Dr. Glasgow worked with others to computationally design proteins with new functions, such as sensing and responding to other molecules in the environment. But the interactions they worked with in these studies involved inter-molecular binding, not the conformational changes that Dr. Glasgow wanted to probe for their ability to elicit new protein functions.
Designing the conformational landscapes of proteins for that purpose is on her to-do list for her new lab at Columbia. One current project focuses on acquiring the detailed protein data needed for predicting and using allosteric effects for designing new proteins. Other investigations center on tailoring proteins for novel tasks–whether that’s neutralizing an emerging pathogen or manufacturing a new cancer drug. Another line of research is all about repurposing that type III secretion system from her grad-school days. At Columbia, her aim is to redesign the system into a protein-delivery device that could, for example, reprogram immune-system cells for anti-inflammatory therapies.
Dr. Glasgow also is working to make science more accessible. As the daughter of Muslim Pakistani immigrants, she has endured her share of frustrating and discriminatory experiences along her scientific journey.
“The access to the education I have had is a huge privilege, but too many people have been excluded,” she says. That’s why she spent Friday nights during her postdoc at San Quentin State prison teaching algebra to incarcerated students with the Prison University Project (now Mt. Tamalpais College).
“My incarcerated students were motivated, prepared and high-performing,” she says. “The scholarship of incarcerated and other historically excluded people is desperately needed in the molecular sciences, so that these communities are empowered to solve the problems that impact them, from the bottom up.”
“It’s important to me that what I am doing can be useful,” stresses s Dr. Glasgow. For her, that usefulness extends from science, engineering and technology development to making sure a life in science and problem-solving is a genuine opportunity for anyone.